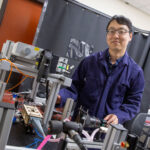
Making sense of disorder in hyperuniform materials
ASU and Princeton researchers collaborate on Department of Defense MURI grant investigating hyperuniformity
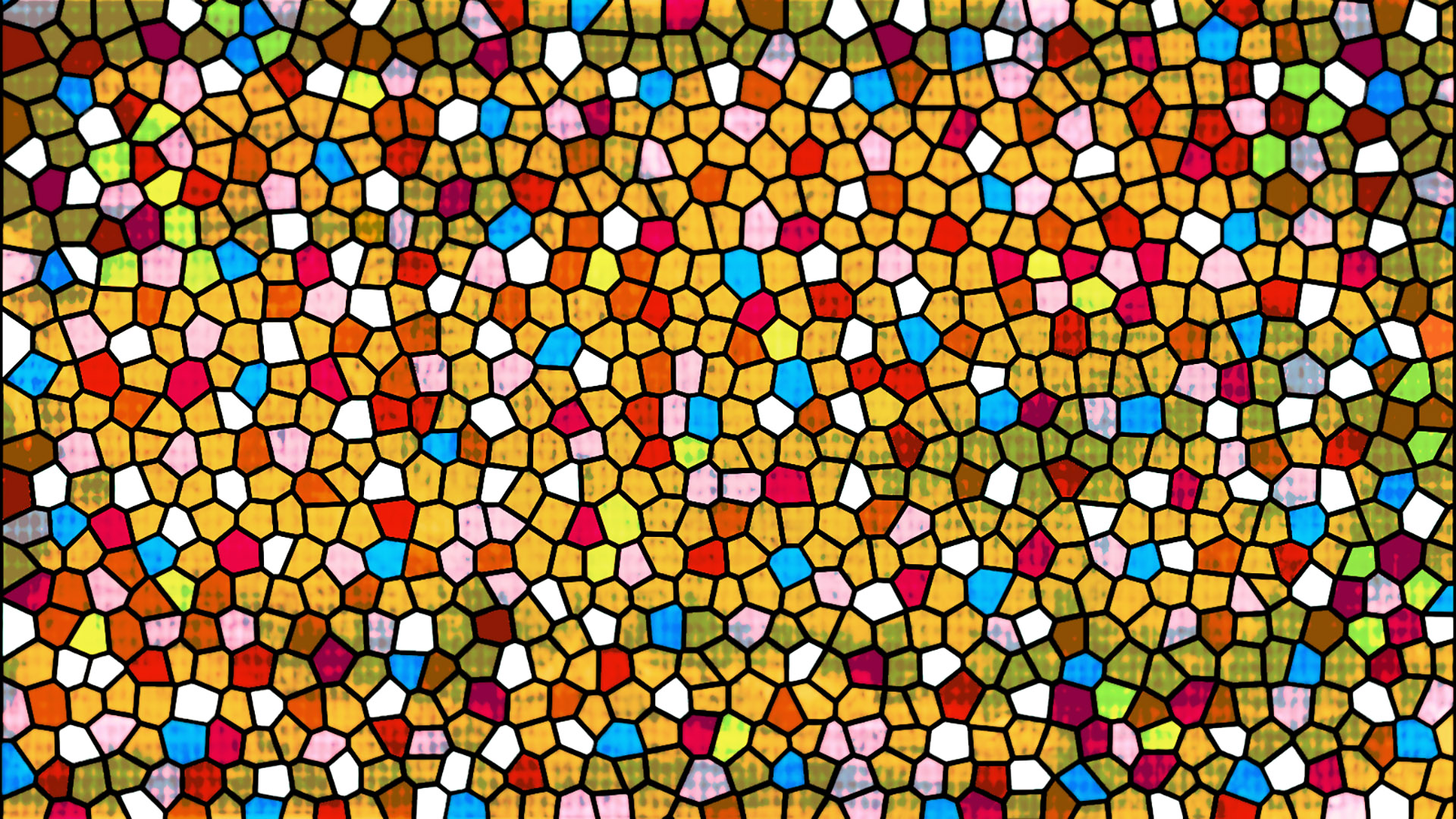
The world is composed of solids, liquids, gasses and plasmas: the four forms in which all matter in the universe. At high concentrations, such as in tiny groups of atoms, matter has different “condensed” states represented by different formations, like ordered crystal patterns, random patterns, and those that lie somewhere in between. One of those in-between states is called hyperuniformity, and its disordered nature contributes unique properties.
Yang Jiao, an associate professor of materials science and engineering in the Ira A. Fulton Schools of Engineering at Arizona State University, is a co-principal investigator on a new Multidisciplinary University Research Initiative, or MURI, project funded by the U.S. Department of Defense to investigate disordered hyperuniformity and its applications. The project, “Transport in Disordered Hyperuniform Systems and Networks,” is led by Salvatore Torquato, a professor of chemistry at Princeton University.
The five-year grant of just over $6 million reflects the DOD’s investment in interdisciplinary research for areas of strategic importance. In 2022, the department has awarded $195 million to 28 research teams spanning a variety of science and engineering disciplines.
Deeper knowledge of hyperuniformity could spark innovation in the field of photonics by providing new and more effective materials to transport light. Understanding hyperuniform networks can also improve communication and transportation networks, as well as our understanding of disordered networks in general, leading to new concepts for designing networks that can adapt to disorder and be highly robust against attacks.
An introduction to hyperuniformity
Research into states of matter has continually advanced over the past 100 years. In the 1950s, scientists understood formations of atoms in two ways: an ordered formation called “crystal” and a disordered formation dubbed “glass.”
Materials scientists discovered a third pattern in the 1980s that they called the “quasicrystal” pattern, which was not random but not completely ordered and uniform like the crystal pattern in that they lack long-range translational order.
Then, in the 2000s, Torquato and Frank Stillinger, a theoretical chemist at Princeton University, discovered a new state of matter — the hyperuniform structure — that looks like a random pattern on local scales as in disordered glass structures, yet possesses unique hidden long-range order on large scales as in crystal structures.
Hyperuniform structures can be found throughout the natural world, for example in birds’ eyes. Birds have great vision due to the wide variety of photoreceptors in their retina.
Other lifeforms with optimized vision typically have photoreceptors arranged in identical patterns, such as in hexagon shapes. However, the variety of photoreceptor cells in birds’ eyes does not allow for an ordered structure. Instead of exact geometric patterns, the hyperuniform system for birds’ vision includes photoreceptors in disorganized patterns of non-identical polygons or organic shapes that optimize the way they can perceive light.
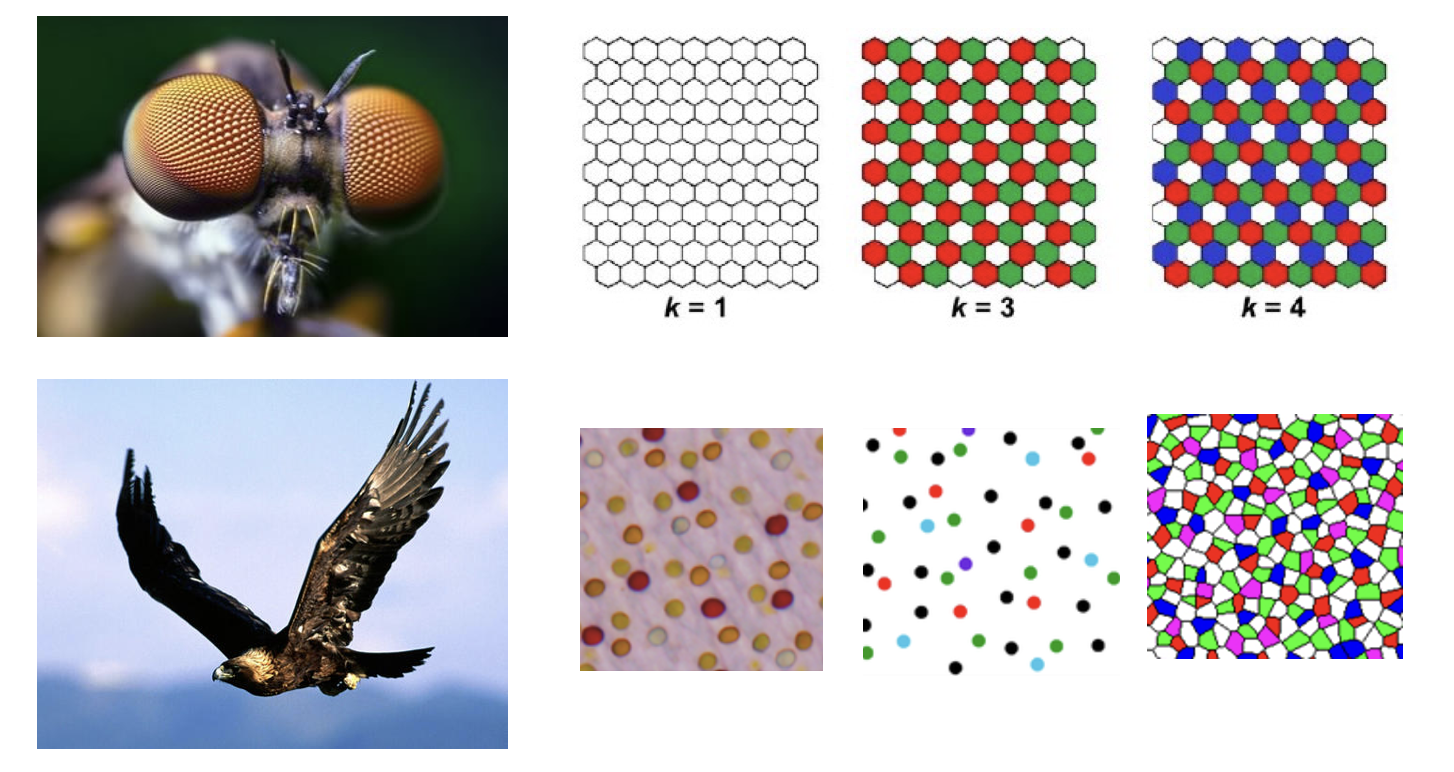
Insect retina demonstrate an ordered or “crystal” atomic structure such as repeating hexagon-shaped photoreceptors, while avian retina have developed hyperuniform structure, which include disorganized patterns of non-identical shapes. Image courtesy of Yang Jiao
The benefits of hyperuniformity
Outside of nature, hyperuniform materials have demonstrated increased proficiency in both electronic and photonic (light or photon) transport.
“Previously, researchers believed that the more disordered a material was, the less effective its electronic transport would be,” says Jiao, a faculty member in the School for Engineering of Transport, Matter and Energy, one of the seven Fulton Schools. “We proved that if the material is in a hyperuniform state, it actually has enhanced electronic transport compared to both crystal and disordered structures.”
When designing a device to interact with electromagnetic waves, it must achieve an ideal “band gap,” a frequency in which the photonic wavelength cannot propagate. Hyperuniform materials have demonstrated a larger bandwidth than other substances, making them ideal materials.
Understanding the network of a disordered system like hyperuniformity has other advantages.
Network theory is a very powerful model for revealing the workings of a variety of different systems, for example, electronic or photonic transport, social networks and the spread of disease. Many of these networks of interest are disordered like the hyperuniform structure. Therefore, using the machinery of hyperuniformity, researchers can better understand the behavior of disordered networks.
“If we develop tools to understand transport in hyperuniform networks, we can immediately adapt or borrow these tools to understand a wide range of transport properties in different materials systems,” Jiao says.
Generating new knowledge of hyperuniform systems
To explore hyperuniform structures, the research team has four “thrusts” or sets of goals for the MURI project. The first of which prioritizes further development of theories using the hyperuniform atomic system so these theories can also be generalized to networks.
Next, the team will produce network-based analyses of hyperuniform systems through graphic representations and network metrics. Researchers will look at generalizations of the hyperuniformity concept in different networks, with focus on topology and graphic representations.
The team will also evaluate information and energy transport in hyperuniform networks by mapping different physical systems in the networks, using the tool developed in the previous thrust to understand physical systems.
Lastly, the fourth thrust will explore the engineering of hyperuniform networks. Once the team understands the tools for the network and are able to apply these tools to understand different physical problems, they can design better materials and networks that meet the goals of the Department of Defense.
As an expert in both hyperuniformity and network theory, Jiao will participate closely in the development of the network-based analyses and the hyperuniform materials.
Outside of his work with this MURI project, Jiao continues to explore the nature of hyperuniformity. He is working in tandem with his colleague Houlong Zhuang, an assistant professor in the School for Engineering of Matter, Transport and Energy, on atomic hyperuniform materials and generalizing the notion of hyperuniformity to quantum states.
“Traditionally, people consider ‘disorder’ as a defect in materials,” says Jiao. “Now, we have demonstrated that disordered hyperuniform materials can achieve nearly optimal properties compared to their crystalline or ordered counterpart. This would suggest a new direction in material discovery and design instead of minimizing defects, make them hyperuniform.”