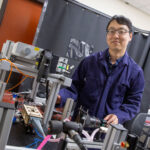
Fulton Schools takes in lion’s share of solar research funding from DOE
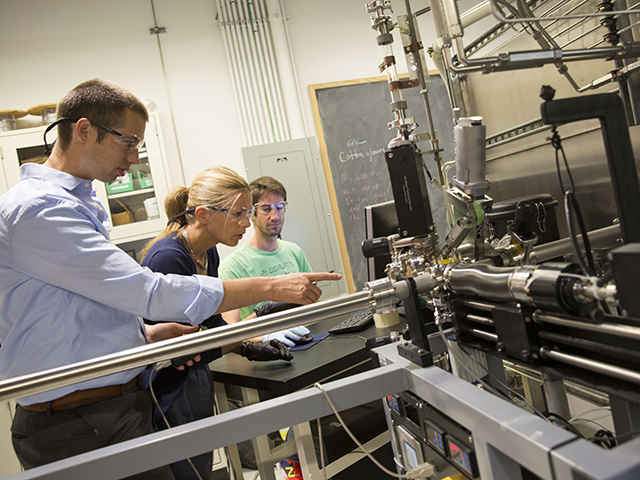
New solar research projects at the Ira A. Fulton Schools of Engineering will receive $3.75 million in funding the U.S. Department of Energy SunShot Initiative announced today. The home of the Engineering Research Center for Quantum Energy and Sustainable Solar Technologies, Arizona State University garnered five of 19 awards, more than any other institution receiving funding. The awards amount to nearly 20 percent of the funds allocated, each with the potential to dramatically reduce solar energy costs.
The SunShot Initiative’s photovoltaics program, which is the focus of the current round of funding, supports research and development projects that lower manufacturing costs, increase efficiency and performance, and improve reliability of PV technologies in order to support widespread deployment of electricity produced directly from sunlight. The PV portfolio includes research directed toward the SunShot Initiative goals as well as critical challenges beyond 2020.
Stacking yields broader solar harvest, reduces costs
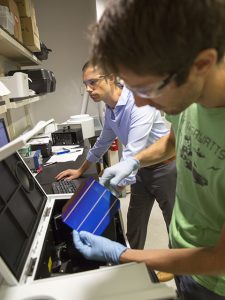
Postdoctoral researcher Mathieu Boccard, front, and electrical engineering Assistant Professor Zach Holman work on solar cells in Holman’s lab. Photographer: Jessica Hochreiter/ASU
In May, a unique collaboration between researchers led by electrical engineering Professor Yong-Hang Zhang and Assistant Professor Zachary Holman wedded two previously disparate solar cell technologies, taking another step toward lower-cost and widely accessible solar power.
The results of their partnership not only broke an efficiency record by a large margin for monocrystalline cadmium telluride cells, but more importantly, also achieved the highest open-circuit voltage ever recorded for a cell of its type.
Now with a new $400,000 award, the partnership between the two research groups will continue, investigating the fabrication of high-efficiency, “stacked” photovoltaic cells, which comprise of two different types of cells. The pair is also collaborating with Wyatt Metzger, of the National Renewable Energy Laboratory in Golden, Colorado.
“The long-term aim of this project is to stack two different solar cells on top of one another, one of which is efficient at converting visible light into electricity and one of which is efficient at converting infrared light into electricity,” explains Holman. “We call this a tandem solar cell.”
By stacking two cells, the projects aims to harvest more of the solar spectrum, with a goal of eventually boosting efficiency of photovoltaic systems by one third. This increase in efficiency could also potentially decrease the cost of installed systems by as much as 20 percent.
Holman, Zhang and their research groups plan to use silicon for the bottom cell, which is widely manufactured and commonly used. While they have an excellent, proven option to convert infrared light into electricity, fabricating a top cell may prove to be more difficult.
Holman notes that existing materials with top cell potential are “either efficient and expensive — only used in satellite technology — or they’re inexpensive but very inefficient.”
In pursuit of a cheap and efficient top cell that absorbs visible light, Holman is utilizing a material system based on CdTe, a continuation of his record-breaking work with Zhang. However, the duo’s previous successes leveraged monocrystalline CdTe, a high-quality, expensive version of the material, unfit for mass commercialization due to its cost.
“This is where Metzger comes in: He’s an expert on the polycrystalline material, or the cheap version of it,” says Holman. “So we’re going to be both pushing the efficiency limits here at ASU and make a manufacturable version of the top cell at NREL.”
Holman also hopes to hire a postdoctoral student to send to NREL to work with Wyatt on the project.
But it was current ASU researchers who Holman credits with the success of the previous collaboration: Mathieu Boccard, a postdoctoral researcher, and doctoral students Jacob Becker and Yuan Zhao in Zhang’s research group.
“Those three really made it happen,” says Holman. “I would say it was due in large part to our existing collaboration that we were able to get this award. And if this project is successful, it will be because of Zhang’s group and our ability to continue to work together well,” he adds.
Modular thinking may lead to cheaper, more efficient and reliable solar power
While Holman’s top cell research is in its very early stages, another project supported by SunShot may have a much more immediate impact on the industry.
“In this project, we’re working at the module scale, or product scale, if you will,” says Holman of the project. “We’re trying to figure out how to take good, commercially-manufactured silicon cells and do a better job of turning them into a module that is not only efficient and cheap, but reliable.”
The $800,000 award supports research to lower the cost of photovoltaic electricity generation in less than five years by developing a module comprised of back-contact silicon solar cells. While such cells commonly feature interlocking metal fingers on their reverse sides and no metal on their face, the researchers have chosen to eschew metal componentry entirely and instead utilize a “flex-circuit” to connect the cells. The flex-circuit is made of two layers of aluminum foil separated by an insulated layer.
By doing so, there is a reduced use of expensive silver or copper in the modules, which eliminates the need for failure-prone solder points. This will increase the reliability of the module, dramatically reducing the cost of modules and the electricity produced.
“Right now, modules come with a 25-year warranty,” explains Holman. “To calculate the cost of electricity from a solar module, you basically take how much electricity it will put out over 25 years divided by how much it cost. If you were to make a module that had a warranty of 40 years, it would cost roughly the same amount, but produce the electricity over a longer period of time. Just like that, the cost of solar energy would go down by a considerable amount.”
If the project is successful, Holman estimates the cost of rooftop photovoltaic energy generation could drop to just four cents a kilowatt hour. Currently, the average cost rests around 10 cents per kilowatt hour.
Holman is collaborating with Mariana Bertoni, assistant professor of electrical engineering, and Govindasamy Tamizhmani, associate research professor at the Polytechnic School on the project. He’s also formed a relationship with NREL to model the project cost to determine market viability. In addition, solar company Natcore Technologies is collaborating on the research.
Holman attributes the project’s core idea to long-time solar industry veteran Charlie Gay, who recently stepped into the role of director at the SunShot Initiative. Gay previously served as president of Applied Solar at Applied Materials Inc., a semiconductor, solar and display support services provider.
“He’s helped my group in many ways over the past two years, and he ended up coming to me with the idea for solar module manufacturing,” recalls Holman.
Reflecting on both awards, Holman notes both tie into larger research goals he’s established for himself and his group.
“I have tried to position my group as the go-to group for silicon-based tandem solar cells and modules, at least in the U.S.,” says Holman. “I’ve been committed to this topic for about two years now and I’m really excited to get started on the work that needs to be done.”
Predictive Physical Modeling improves reliability of thin-film photovoltaics
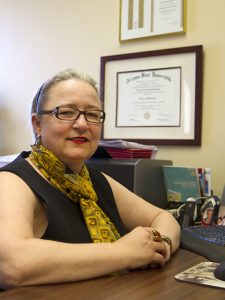
Dragica Vasileska
A key DOE goal for innovative solar research is to overcome current technological and commercial barriers for CdTe solar cells — a photovoltaic technology based on the use of a thin semiconductor layer that absorbs and converts sunlight into electricity.
However, TFPV modules often suffer from performance degradation over time — some of the changes are reversible and some are not, which makes deployment, testing, and energy-yield prediction more challenging. While there are multiple hypotheses that suggest the causes of degradation of CdTe solar cell devices, none of them have received strong theoretical or experimental confirmation.
Dragica Vasileska, a professor of electrical engineering and a senior sustainability scientist with the Julie Ann Wrigley Global Institute of Sustainability, has received $813,000 in funding for research that focuses on improving the reliability of CdTe and other TFPV by identifying the causes and solutions to degradation issues.
Vasileska and her team are developing a self-consistent software tool that aims to eliminate the ambiguity between observed solar cell changes under stress and their physical root cause, allowing them to more accurately interpret performance and material properties of CdTe and other TFPV devices.
The software includes a modeling tool that accounts for atomic diffusion and drift, and can also account for electronic behavior and device performance, thus allowing researchers to simulate recombination losses over time in thin film absorber materials under specified process and stress conditions.
In addition, the team will produce advanced models describing capture/emission/recombination phenomena relevant to multivalent dopants, amphoteric centers, and donor-acceptor pairs. These models, incorporating grain bulk, grain boundaries, and hetero-interface properties, will be implemented in a self-contained simulation tool that will drastically reduce interpretation ambiguity and, for the first time, allow for predictive design of TFPV devices.
“The innovative aspect of the research is in understanding metastability and in improving reliability of CdTe and other thin-film PVs through modeling and simulation based on a unified solver,” explains Vasileska. “The solver will provide infrastructure development for predictive polycrystalline device behavior and will replace empirical trial-and-error with a more productive engineering by design approach.”
Pushing the limits of silicon heterojunction solar cells
About 80 percent of solar cells are manufactured using aluminum back surface field technology, which has reached the limit of cost-reduction opportunities. One of the most probable candidates for mass production replacement is silicon heterojunction (SHJ) technology — a more advanced manufacturing process that has achieved high efficiencies in the lab.
The group at ASU Solar Power Laboratory, led by Stuart Bowden, a research professor in the School of Electrical, Computer and Energy Engineering and a senior sustainability scientist for the Julie Ann Wrigley Global Institute of Sustainability, has been developing a manufacturing technology for SHJ cells since 2012, when an SHJ pilot line was established.
As a seed project from the QESST Engineering Research Center, the line already has a capability of weekly processing about fifty SHJ cells on full 5” and 6” wafers with 21-22 percent efficiency.
This new project, which has received $837,000 in funding, examines the manufacturability of n-type industrial SHJ cells and develops methods to improve yield and increase the attractiveness of this type of cell to manufacturers.
The research performed will help to improve cell efficiency by two percent and reduce the cost of the cells by improving electrical yield based on a range of new processing improvements. The project also is working to demonstrate the feasibility of using thinner cells to increase the lifetime of the wafer and achieve a 26 percent record efficiency. The knowledge gleaned from this research is expected help to improve the manufacturing of SHJ cells.
A major challenge of SHJ technology is the large efficiency difference between the record laboratory cells and the cells produced in high throughput industrial lines. Electrical recombination losses not only limit the efficiency of the cells, but also lead to a wider efficiency distribution and higher electrical yield losses. The losses are caused by numerous factors, including variability of the quality of commercial silicon wafers and imperfections of surface passivation occurred during wafer processing. The main goal of this project is to study electrical recombination losses in industrial SHJ cells and develop methods to mitigate the losses in high volume production. The project will collaborate with Sinton Instruments, a leader in characterization of silicon solar cells, as well as a number of undisclosed silicon wafer manufacturers.
According to Bowden, this project will allow ASU Solar Power Laboratory to focus for the first time on the improvements to the industrial SHJ technology with immediate transfer to industry.
“SunShot funding will bring together the advances in processing of SHJ cells that we have developed in the last three years,” Bowden said. “We expect that mitigation of recombination losses in industrial SHJ cells will increase their efficiency to more than 23 percent and ultimately lead to lowering PV electricity cost below six cents per kilowatt hour. We will also investigate applicability to a wide range of silicon wafers and very thin silicon wafers to further reduce costs. Last but not the least, reduction of recombination losses can allow us, for the first time, to demonstrate a small area laboratory silicon cell with 26 percent efficiency.”
Thermally Conductive Backsheets (TCB) For Reduced Operating Temperatures
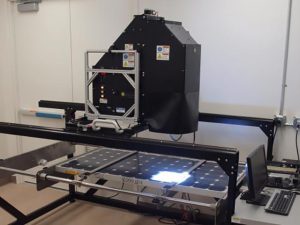
Equipment used to test current and voltage to measure performance of solar cells within a solar panel in Associate Research Professor Govindasamy Tamizhmani’s Photovoltaic Reliability Laboratory on the Polytechnic campus. Photo courtesy of Govindasamy Tamizhmani
When it comes to solar power, more sunlight equals more power, right? Not exactly.
On bright, sunny days solar modules actually operate around 30 degrees Celsius higher than the ambient temperature. The higher the operating temperature, the lower the performance — commonly used crystalline silicon modules can lose as much as 30 percent of the rated power on a hot day.
Govindasamy Tamizhmani, associate research professor at the Polytechnic School, aims to mitigate this age-old drawback of solar power production with an innovative use of thermally conductive backsheets. Solar module manufacturers commonly use thermally conductive backsheets as a packing material, but Tamizhmani believes they have potential to reduce the operating temperature of a solar module.
“A reduction in operating temperature of the [photovoltaic] modules not only increases the power production but also increases the lifetime in the field,” says Tamizhmani.
In pursuit of these goals, Tamizhmani has been granted a $900,000 award to research the feasibility of using backsheets to boost performance and reliability of solar modules. The research aims of this award tie directly into the ongoing work at Tamizhmani’s Photovoltaic Reliability Laboratory, where he serves as the director. This marks the second SunShot award Tamizhmani has received this year, the first being an $800,000 award beginning in August 2016 to correlate lab accelerated photovoltaic module degradation with modules degraded in the field. This way, researchers will be able to more accurately predict a module’s lifetime.
Some solar modules employed active cooling solution in the past to reduce operating temperature, pumping water through pipes to cool them on the back, but Tamizhmani believes this method is too costly to reap any notable benefits. By reformulating an existing material, Tamizhmani hopes to improve power production, reduce degradation and increase the lifetime of solar modules.
Tamizhmani is collaborating with Electric Power Research Institute and Sunset Technology on the project, which he believes could “immediately become a viable part of our energy portfolio” if successful.
Media Contact
Terry Grant, [email protected]
480-727-4089
Ira A. Fulton Schools of Engineering